In Summary
- Although limited, evidence suggests that when applying water to crops, ensuring the water doesn’t contact the edible parts of the crop reduces microbial risk. With the least risky being subsurface irrigation through to the most risky, overhead irrigation.
- In addition, the type of crop, and type of pathogen will impact the level of crop contamination, as different pathogens attach to fresh produce using different mechanisms.
- Hydroponics systems can also be reservoirs of contamination. Experiments found irrigation water contaminated with Salmonella can penetrate the root of tomato and travel into the plant tissue. With risk increasing the greater the initial water contamination was.
- Pipes used to transport water can also harbour microbes, with E. coli found to be capable of growing in pipes between irrigation events.
- Soil splash from rainwater can also transfer soil, and bacteria, onto the under surface of crops grown outdoors.
- Internalisation of human pathogens into crops can occur and persist for extended periods. Internalisation can occur through use of contaminated water during seeding or watering, as well as invasion into openings on the plants on many surfaces.
Application of irrigation water and crop contact
Although there is not a huge body of scientific evidence available currently, there is enough to say that the method of application of irrigation water to fresh produce has an effect the microbiological risks associated with the crop. In general, and as might be expected, keeping water away from the edible parts of ready to eat crops (crops that are consumed without cooking), results in a lowered risk of an outbreak of foodborne illness. Thus, when choosing which irrigation method is most appropriate for each particular crop; consideration should be given to the location of the edible part. There is a large and expanding volume of scientific evidence that shows abstracting surface water during rainfall increases risk because surface water during rainfall has elevated bacterial numbers contained within it (Kaushik et al 2014; Rodrigues et al. 2020; Balleste et al. 2020; Lee et al. 2020; Lizaga et al. 2020; Steinfeld et al. 2020). For those crops which grow above ground with a little clearance (e.g. salad onions, lettuce); Figure 1 below shows the most and least risky irrigation methods.
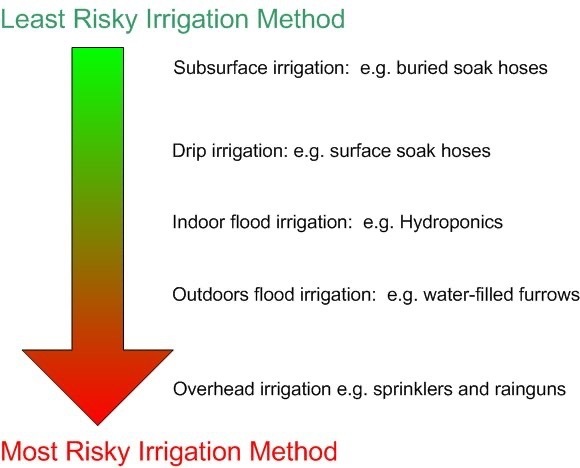
(Collated from information provided by Steele and Odumeru 2004; Song et al., 2006; Critzer and Doyle, 2010; Nnadozie and Odume 2019; Rodrigues et al. 2020 and Weller et al., 2020.)
In addition, to keeping water away from the edible parts of crops, a number of publications have highlighted the importance of attachment in the contamination of fresh produce (Song et al., 2006; Critzer and Doyle, 2010). In simple terms, zoonotic agents (that is, pathogens from animals that have the potential to infect humans) have an ability to recognise and glue themselves to the surface of some (but not all) types of fresh produce. Different pathogens are better at attaching to different crops. Investigation of attachment and the generation of lists describing what types of fresh produce are most susceptible to which pathogens, is currently in the very early stages. The results of those few published experiments describing the application of irrigation water to various produce are summarised below.
Flood irrigation versus subsurface irrigation
Song and colleagues (2006) compared irrigation undertaken by flooding furrows with a subsurface drip system to see which had the best microbiological safety. In their experiment, melons, lettuce and bell peppers were grown as crops. The water used for irrigation was deliberately contaminated with E. coli, Clostridium and a bacterial virus. Over a 14 day period, the numbers of these microorganisms were followed on the crops, soil surface and also in the subsoil (10 cm depth). Overall, greater contamination was recovered from the furrow-based system when compared with subsurface drip. However, the decline of the microorganisms was fastest on phylloplanes (the surfaces of leaves) and the soil surface. The microorganisms which were delivered below the soil surface died off most slowly. For the produce tested, Song and colleagues concluded that “subsurface drip had a great potential to reduce the health risks associated with the use of contaminated irrigation water.” The implications for underground crops such as carrots were not investigated by Song, but clues to the fate of zoonotic agents in ground crops are available.
A study which investigated the fate of Salmonella in soils (NB not contaminated with poor quality irrigation water) used to plant radish, turnip, and broccoli found there was a higher prevalence of contamination as compared with lettuce, tomatoes, and carrots grown in the same contaminated soils (Barak et al., 2008). Salmonella contamination of lettuce and tomatoes was exceptionally low, suggesting that the strains of Salmonella used by the researchers do not readily attach, grow, or are attracted chemotactically (chemically attracted) to the chemicals released by the roots of these crops.
Contamination of above ground produce by sprinklers
Hutchison et al. (2008) determined the implications of using contaminated irrigation water applied by sprinkler onto newly planted baby spinach and lettuce on two different soil types in the UK. The fate of the pathogens (E. coli O157, Campylobacter, Listeria monocytogenes and Salmonella Enteriditis) was followed on the produce and in the soil over several different growing conditions over time. The experiments showed that overhead sprinklers can contaminate produce grown above ground. Later work by Mootian et al. (2009) investigated the transfer of low numbers of E. coli O157 to crops from manure amended soils and contaminated water. The authors concluded that transfer occurred, but did not investigate mechanisms of transfer. Further studies by Fonseca et al. (2011) confirmed that bright sunshine could rapidly reduce numbers of E. coli. In Arizona, Fonseca et al. (2011) determined that E. coli applied to crops using overhead sprinklers was not detectable after 7 days. The Fonseca study also reported that water applied to crops using furrows increased the soil survival of E. coli.
However, other researchers have shown persistence for almost 6 months during a winter growing period (Islam et al., 2004). Oron (2002) estimated that if drip irrigation was used to apply contaminated irrigation water, the microbiological risks of a food disease outbreak were 100-1000 times lower than if the same water is applied by overhead sprinkler. In a systematic review of risk factors for produce contamination, Park et al. (2012) concluded that spray irrigation using contaminated water was one of the highest risks for causing contamination of the underside of leaves.
Hydroponic systems
The implications of using contaminated water in hydroponic crop rearing was investigated by Guo et al., 2004. Colonisation of tomato plants by Salmonella was used as the model of the study. After nine days of exposure of the tomato plant roots to a solution containing large numbers (over 10,000 cfu of Salmonella/ml) of bacteria, isolation of large concentrations of Salmonella (more than 1,000 /g plant tissue) was observed. The isolations were from the roots and the developing cotyledons (leaves and shoots). The plants were too young to have developed fruit. Although the study showed there is the potential for a major outbreak from a hydroponic system if large scale contamination occured, the numbers of bacteria used in the experiment were exceptionally high, and arguably too high to represent a credible real-life scenario.
The original studies of Guo and colleagues were confirmed and extended by Koseki et al (2011) who compared E. coli O157:H7, Salmonella, and Listeria monocytogenes applied to either roots or leaves in hydroponically grown spinach. The hydroponic solution was contaminated with either a low (103) or high (106) concentrations. In parallel, the human pathogens were inoculated onto the growing leaf surface, at concentrations of 103 and 106 cfu per leaf. In keeping with the findings of Gou et al (2004), contamination was observed only through the root system and at the higher concentration. For all the pathogens assessed, plant colonisation was rare when the lower concentration was used. Compared with the roots, contamination through the leaf occurred infrequently even when the contamination level was high. Statistical analyses of the Koseki results showed that the risk of contamination from the roots was 7 times higher than the risk of contamination from the leaves. In addition, the risk of contamination by L. monocytogenes was 30% of the risk for Salmonella and E. coli O157:H7.
One property of hydroponic systems not assessed by either of these studies was that hydroponic water contains nutrients which can be utilised by bacteria. Water management is a critical part of such systems. Although the primary supply is drinking water quality, there needs to be in line treatment to maintain acceptable water quality. There is currently very little scientific information regarding this aspect of hydroponic production.
Water transport pipework
Pachepsky et al. (2012) investigated the influence of irrigation water pipework to irrigation water quality. The focus of the paper was growth on the internal surfaces of the pipework and in the water remaining inbetween irrigation events. Small squares of stainless steel were placed inside the pipes used to transport water to a sprinkler irrigation system for a week in-between irrigation events. Untreated water from a supply creek, sprinkler water, residual water left in the pipes from the previous irrigation and biofilms on the steel squares were tested for numbers of E. coli. High numbers of E. coli were found in the water remaining in irrigation pipes between irrigation events, suggesting growth of E. coli in the pipes. However, the numbers of bacteria growing as biofilms on pipe walls (as assessed from the steel squares) was estimated to be higher than in water in the pipes. The report authors recommended flushing of the irrigation system prior to irrigating as a way to reduce the risk of microbial contamination of produce. Dead legs and redundant spurs in pipework are also areas that can allow bacterial growth in pipe water.
An area of increasing concern is antimicrobial resistance (AMR) in pathogens such as E. coli O157. The issue relates to people who become ill after eating contaminated food and the inability of medics to treat their infections with antibiotics if the infective agents are resistant to antibiotics. Blaustein et al. (2016) investigated pipe-based irrigation systems that distributed surface waters to determine if antibiotic-resistant bacteria growing as biofilms inside transit pipes were an issue. Multi-drug resistance (MDR) was observed for all bacterial groups, most often for the faecal coliforms. A key finding of the study was that the types of antimicrobial resistance (AMR) present changed from week to week. AMR bacteria grow in and are readily isolated from pipes used to transport surface waters.
Water and soil splash
Although it is best practice to keep irrigation water away from the edible parts of the crop, there are a number of other factors that need to considered in conjunction with water-crop contact. The first is that pathogenic bacteria and other microorganisms survive longer on (and even longer within) soil when compared with crop phylloplanes (i.e. the surface of a crop). If there is a possibility that heavy rain can fall directly onto fields containing crops, then soil splash should be considered when assessing risks. Soil splash can transfer pathogens from the soil surface onto the surfaces of crops as shown in Figure 2 below.
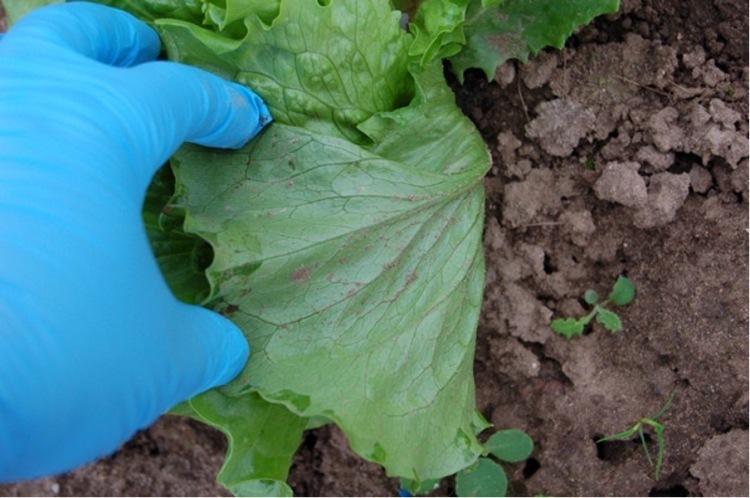
AHDB Horticulture have funded work (Monaghan and Hutchison, 2012) which has attempted to determine how far pathogens contained within contaminated soils can be transferred by raindrops. Figure 3 below shows an agar strip (agar is used for growing bacteria). Each of the purple spots is a colony of E. coli. The E. coli was transferred to the agar strip from contaminated soil by a single raindrop. A large raindrop (of 50 microlitre volume) can transfer E. coli up to 0.5 metres (20 inches) to the side of the point of impact. During the transfer, bacteria can reach a height of 0.3 metres (12 inches). The smaller the raindrops the less distance that pathogens are spread (Hutchison and Monaghan, 2012)

A single simulated raindrop was allowed to fall from 6 metres onto soil contaminated with E. coli and the splashes allowed to land onto selective agar strips laid in the X, Y and Z axes which allowed the selective growth of the pathogen. The distances travelled by the zoonotic agent were determined by allowing the pathogen to grow to visible colonies which could then be counted.
There were no significant differences with regard to bacterial transfer between the two loamy soil types which were compared in the Hutchison and Monaghan (2012) study. However, Park et al (2012) systematically identified that growing produce on clay soil was a risk factor for contaminated produce, although the nature of the risk was not identified.
Chemotaxis and rhizosphere growth
Habteselassie and co-workers (2010) have undertaken work using E. coli which were genetically modified to allow easier isolation from soil and crops. Introduction of E. coli to soil via manure or manure in irrigation water showed that E. coli could colonise the lettuce rhizosphere. Regardless of whether manure or water was used as the introduction method, 15 days after rhizosphere establishment, E. coli was detected on the phyllosphere of lettuce at 2.5 log cfu/g. E. coli persisted in the bulk and rhizosphere soil throughout the study duration of 41 days, but were not detected on the external portions of the phyllosphere after 27 days. The Habteselassie study is important because it establishes that E. coli can move through soil towards a nutrient source. Furthermore, the roots of plants such as lettuce can leak carbon compounds into the soil and these exudates can be used by E. coli for growth and multiplication. A review of related work by Jacobson and Bach (2012) between 2010 and 2012, has provided further confirmatory evidence that bacterial growth can occur in a number of rhizospheres including tomatoes and spinach.
Internalisation
Another consideration which has created increasing concern for the fresh produce industry in recent years has been the issue of internalisation of human pathogens into crops. An easy to read summary of the literature relating to internalisation has been prepared by Deering et al. (2012).
The important points relating to internalisation are:
- Human pathogens such as Salmonella and E. coli O157 are present in water.
- When seeds are soaked in contaminated water, or contaminated water is used for irrigation or pesticide application or contaminated water is used to wash harvested produce, these human pathogen can invade the plant tissues.
- Invasion can occur through natural openings in the plant such as stomata, lenticels and lateral roots. Invasion can also occur via damaged plant tissue including cuts made to harvest crops.
- Once inside the crop, the human pathogens are protected from harsh environmental conditions and can possibly survive for extended periods. However the insides of plants are not sterile and human pathogens attempting to establish inside a plant must compete with the indigenous bacteria for nutrients and space before colonisation can be accomplished. In many cases human pathogens do not successfully establish inside the plant.
- Internalisation has been described in roots, lateral roots, root hairs, stems, leaves and fruits.
A recent review by Riggio et al. (2019) investigated the risk of internalisation from six types of hydroponic culture. These were nutrient film technique (NFT), deep water raft culture (DWC), flood and drain, continuous drip, the wick method, and aeroponics. The first five approaches were the most commonly used in the production of leafy vegetables. A general conclusion of the review was that each of these systems may confer different risks and advantages in the production of leafy vegetables. The range of different laboratory methods used by the different authors made making conclusion from the review difficult.
Although there has been a lot of work in recent years studying internalisation, it has not yet been proven as the cause of an outbreak of human illness. Furthermore, the numbers of cells which are internalised into plants are always very low, although the Riggio review suggests uptake might be higher from hydroponic systems compared with conventional production. In most cases, internalisation is studied in a laboratory, and the numbers of internalised pathogens are typically below the infectious dose required for illness; even if the entire plant was consumed by one person. At the current time, it remains unclear what role, if any, is played by internalisation in terms of fresh produce food safety in commercial scale production. However, irrespective of the risk of internalised pathogens to human health, fresh produce must comply with relevant legislation, and therefore is still of concern to growers.
Pesticide, fertiliser, and nutrient applications
In addition to irrigation, water can be used to apply pesticides and other chemicals such as NPK and liquid urea fertilisers to crops. A risk assessment was undertaken by Stine and co-workers (2011). Using source water contamination data from the USA, which is broadly comparable with similar data in the UK, a 1:10,000 annual risk of infection for Salmonella and enteric viruses in surface and ground waters could easily be exceeded for some water sources. To reduce the risks associated with the consumption of fresh produce, the authors advise that if surface water is used to prepare pesticides or other crop applied chemicals in spray applications, it should be evaluated for its microbiological quality prior to application.
Decontamination and irradiation
As long as irradiation is not used to cover up poor hygienic practices, health, manufacturing or agricultural practice, is beneficial for consumers and poses no health hazard to them; it is legal to irraqdiate food in the UK. However, it is considered highly unlikely that growers would irradiate fresh produce to ensure it is free from human pathogenic microorganisms. The Food Irradiation Regulations 2009 (near-identical versions for England, Scotland, Wales and NI) set out the requirements for producing, importing and selling irradiated food in the UK. The main issue with treated fruits and vegetables is that they are required to be clearly labelled as being exposed to radiation. The labelling might strongly discourage consumers from buying such hygiene, health or good manufacturing or agricultural practice foodstuffs.
Although unlikely to be of practical use, Puerta-Gomez et al (2013) have undertaken mathematical modelling of the likely outcome of adopting irradiation as a critical control point. The work shows that the spinach is still a highly safe product when cross-contamination occurred during post-harvest washing if the produce was harvested at 20oC, stored for at least 5h, washed with water containing chlorine at 220 ppm, and then exposed to a 1 kGy dose of radiation. 220 ppm chlorine is unlikely to be used to wash crops because there are now legal limits on chlorate concentrations in foods introduced in 2020.